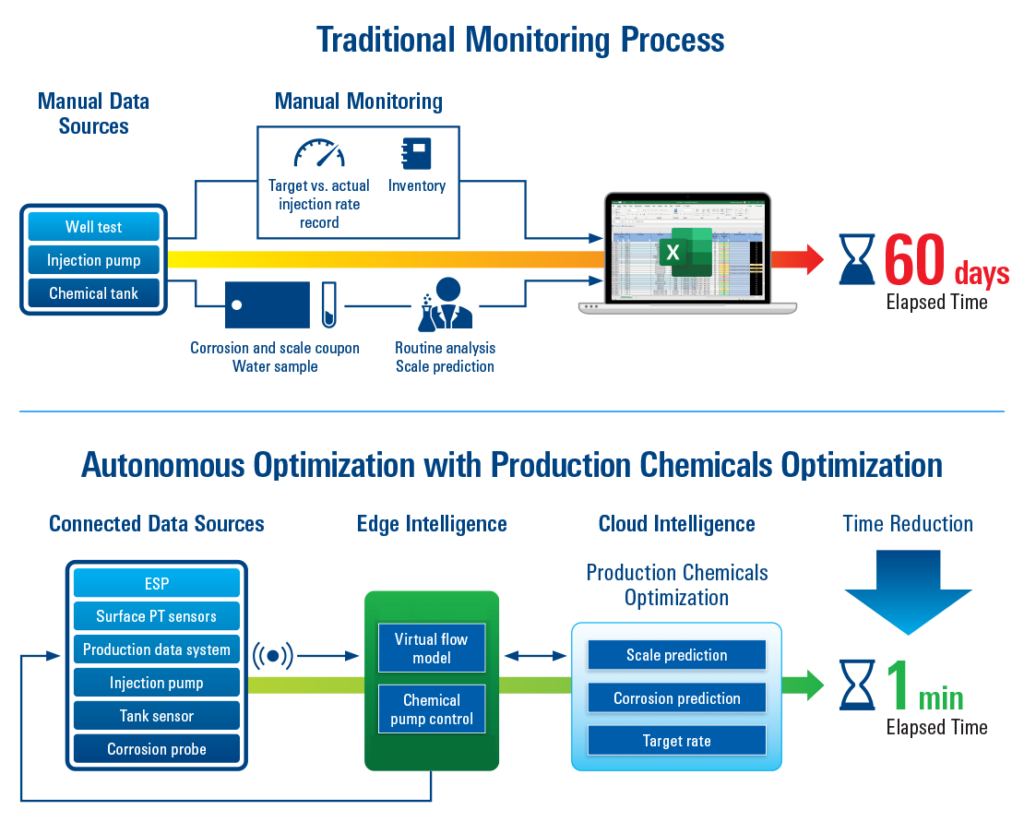
Producers find a considerable amount of their operating expense (OPEX) comes from managing risks associated with corrosion. Monitoring and chemical adjustment workflows are typically manual, and performed at low frequencies, leading to delays in event detection. As a result, the potential for negative events such as production shutdowns and well failures increase. integrating chemistry domain experience with edge analytics, machine learning models, and intelligent equipment can transform manual processes into an autonomous solution. This solution can easily be deployed in an oilfield challenged with a high number of electric submersible pump (ESP) failures due to corrosion and scale that resulted in significant production losses and unforeseen workover costs. The designed digital architecture supports autonomous management of scale and corrosion through remote monitoring and automated chemical injection. Real-time data is acquired from connected equipment, processed in an edge device running artificial intelligence, and autonomously sent to chemical pumps. Data from sensors, connected devices, and models are visualized in cloud applications, or integrated into existing client systems for end user analysis and full visibility of the entire process. The main technical objectives are highly accurate models, precise chemical injection, and a reduction of well failures which ultimately leads to optimized operations, reduced well failures and workover costs, and maximized production.
Background
- System Survey: This requires a full review of the basic production chemistry of the system and includes data on the processing equipment, design, and materials of construction. It also includes data on the composition and physical and chemical properties of the oil, gas, and water phases that are being handled. For an existing field the survey, current and historical performance as well as any specific reports of system malfunction or unexpected observations are also included.
- System analysis: This is required to get a full understanding of how the production chemistry changes throughout the process. For many systems, this will include the modeling of various scenarios.
- Solution identification: This may involve testing a variety of existing production chemicals either under laboratory or field conditions and will frequently involve customization or blending of specialized chemicals.
- Solution application: Once the suitable chemical has been identified, it will need to be proven under actual field conditions. Various chemicals can be compared for suitability in a laboratory environment, but only under actual field conditions can the dosage be optimized.
It is important to note that the above processes are executed at a single point in time, using data points representative of the system at the time they were measured. Therefore, all the chemical treatment recommendations are based on a static data set. However, key factors impacting scale and corrosion risk such as temperatures, pressures, and produced fluid rates are dynamic and frequently change as the fluid moves through the well. To understand these changes, routine monitoring of the system is required to determine if the chemical injection needs to be adjusted to maintain protection. Some of the common methods for monitoring changes in these factors include:
- Well test: to monitor for changes in production
- Coupons: to measure corrosion rate, metal loss, scale deposition
- Water analysis: to monitor changes in water chemistry that may indicate metal loss or scale
deposition - Chemical residuals analysis: to determine the presence of corrosion and scale inhibiting residuals
throughout the well’s production system
Challenges of the Traditional Manual Systems
The problem with the common monitoring methods is they are manual and, as such, do not provide updated data at an optimal frequency. For example, in a typical monitoring program, corrosion coupons are exposed for a 90-day duration before being removed for a laboratory analysis. This gives basic corrosionrate measurements at a frequency of four times per year. The weight loss resulting from any single coupon exposure yields the “average” value of corrosion occurring during that exposure. The disadvantage of the coupon technique is that if a corrosion upset occurs during the period of exposure, the coupon alone will not be able to identify the time of occurrence of the upset and, depending upon the peak value of the upset and its duration, may not even register a statistically significant increased weight loss. Potentially compound the problem is the fact that chemical adjustments are made based on these data, with the data being so infrequent, it can lead to extended periods between monitoring when the chemical injection rate is either below or above the optimal rate, in many cases leaving the well unprotected.
- Data availability: updated well test data, temperature and pressure changes, and chemical composition data are available at a maximum frequency of once per month.
- Manual chemical adjustments: once new data is available that may necessitate a change to the chemical injection rate, a field engineer must drive to the well location and manually adjust the chemical injection pumps.
- Lack of visibility of chemical injection systems: chemical injection pumps can go down or lose efficiency for several reasons such as mechanical failure or factors such as gas accumulation in chemical injection lines. Because there are hundreds of chemical pumps, and the wells are often in remote locations, issues with the chemical injection may not be known for several days in many cases.
Developing an Intelligent Solution
The steps to move from the traditional manual corrosion and scale monitoring methods, toward a proactive approach are:
- Understand which data was required at a higher frequency. For corrosion, it was determined that the two key data required were produced water rate and corrosion rate. For scale, produced water rate, pressure and temperature changes were determined to have the largest impact on the risk of scale precipitating.
- Determine which of that data could be made available by digitally connecting existing equipment. Because these wells use ESPs for artificial lift, we can digitally acquire downhole pressure and temperature data by connecting directly to the ESP controller. Connecting to existing wellhead temperature and pressure sensors provided the surface data.
- Identify where predictive models could be implemented to bridge data gaps. Flow rate, corrosion risk and scale precipitation risk were identified as the variables needed. Edge applications developed in an IIoT device helped manage the whole modeling cycle: data gathering, model building, calibration, and results. These Edge applications include a Virtual Flow Meter (VFM), Scale Prediction, Corrosion logic and chemical injection control algorithms. All of these are running in real-time.
- Procure and install any additional digitally enabled equipment required.
- Develop ingestion methods to incorporate data stored in the customer’s internal systems. The customer stored water chemistry analysis results and well test data in their production data management system (PDMS).
- Process data as close to the equipment as possible and implement a closed loop control system. This is a key step in the process as it is where the project makes use of the data being generated by the connected system and utilizes it to activate the chemical injection system. A recommended chemical injection rate will be produced and if it is different from the current injection rate, it will be presented in the cloud application as an insight that could be approved manually or autonomously, and the new injection rate setpoint fed through the IIoT gateway, to the chemical pump controller.
- Develop a cloud based interactive visualization application to provide end user full visibility and remote-control capabilities. This allows authenticated users to log into a secure and collaborative environment that enables visualization of real-time data from connected equipment, results of Edge processed calculations, and data ingested from the customer’s PDMS. Additionally, this is where automatically generated insights regarding changes in flow conditions and resulting chemical injection recommendations can be viewed and approved either remotely or autonomously.
The task of transforming this workflow into a real-time solution requires real-time data. Most of it is available directly from field instrumentation or data-driven and physics-based models that generate information and insights at a higher frequency.
Conclusions
- The acquisition of real-time input data through connected equipment and model-based simulations enhances understanding of an optimal chemical injection rate in real time.
- Results indicate traditional infrequent and manual monitoring methods may seem less expensive on the surface. However, to achieve sustainability targets and reduce total operating costs investing in a digitally enabled real-time corrosion and scale control system may well prove to be a more effective approach.
- Significant value can be realized by leveraging IIoT based infrastructure to consolidate all the data required to monitor the effectiveness of the chemical treatment program in a single collaborative environment.